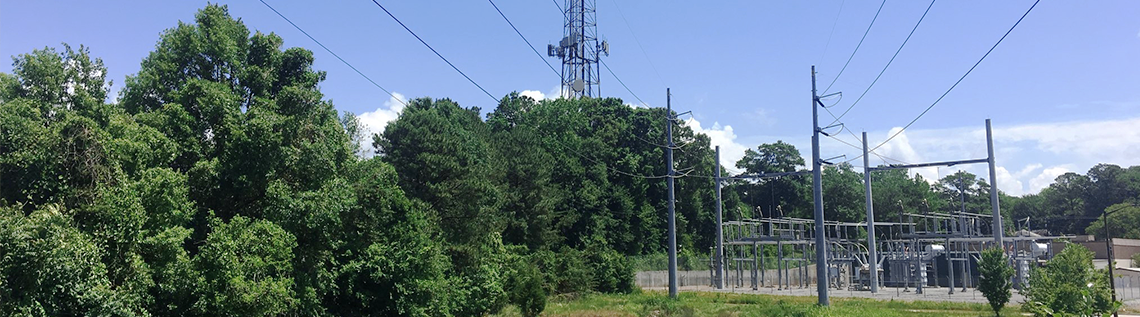
Seeing what people working for real organizations have done out in the real world is sometimes more helpful than looking at hypothetical simulation examples. This may especially ring true for those of you in the power industry, where design failures and other missteps can have a grave impact on customer satisfaction as well as the bottom line.
With that in mind, here are 10 real uses of the COMSOL Multiphysics® simulation software in the power industry.
1. Predict and Minimize Noise in Transformers
Transformers are great for safely connecting high-voltage lines with local power substation, but they’re not silent — and just as high voltages are dangerous, so are high levels of noise. ABB Corporate Research Center (ABB CRC) found that simulation is effective for developing new and optimizing existing power transformer equipment with noise regulations in mind. In particular, they needed to analyze the inner workings of their transformers, because the noise stems from both the core and the oil tank.
Additionally, to produce simulations that accurately represent the real world, the team at ABB CRC had to couple electromagnetics, acoustics, and structural mechanics in a single environment. That’s why they turned to COMSOL Multiphysics. Now, the team is able to optimize their transformer designs with minimal hum before manufacturing them.
Left: A transformer made of a metal core with coils of wire wound around different sections, protected by an enclosure, and an insulating oil inside. Right: Acoustic analysis of the sound pressure field around the core and transformer.
Read the full story here: “From Spreadsheets to Multiphysics Applications, ABB Continues to Power Up the Transformer Industry”
2. Plan Underground Cable Networks Accurately and at Low Cost
Safe. Reliable. Affordable. Managing the electric grid involves meeting all of these requirements. National Grid, which services homes and businesses in England and Wales, needs accurate cable ratings when planning where to lay new cable, especially in older sections that have been repaired using different materials in the same cable line. Managing underground cable networks requires a deep understanding of how surrounding soil, cable age, repairs, and proximity of other cables will affect performance.
However, testing these high-voltage systems is difficult due to their enormous size and the fact that cables have to be analyzed in context rather than in isolation. Finite element analysis (FEA) is up to the task: By modeling cable components with COMSOL Multiphysics, National Grid can ultimately make good decisions about where to lay new cables and how to best repair old ones — thereby minimizing installation costs.
Left: A section of underground cables. Right: A model showing the normalized airflow profile of the cross section of a tunnel containing high-voltage cables.
Read the full story here: “National Grid Models Underground Cable Routes”
3. Manage the Grid More Efficiently
Cable health assessment is vital to keeping the electric grid running smoothly. In addition to using traditional testing equipment (such as infrared, ultraviolet, and partial discharge), the assessment process has to account for cable structure and material, impurities in the cable, voltage fluctuation, and operating conditions and environments. Multiphysics simulation leads to more accurate cable health assessments, but how do you get these simulations out in the field where the health assessments take place and repair decisions are made?
At Wuhan NARI Group Corporation (NARI) of the State Grid Electric Power Research Institute in China, the answer to this question is to turn multiphysics models into simulation applications and give them to the field technicians. The Application Builder in COMSOL Multiphysics allows simulation engineers to wrap sophisticated models in easy-to-use interfaces with limited input fields. Armed with a custom application that NARI calls the Cable Condition Analysis Expert System, field technicians can then assess cables confidently based on accurate simulation results generated from simple data entry.
Left: The insulating layer of a cable showing mechanical damage. Right: A simulation application for assessing cable health, called the “Cable Condition Analysis Expert System”, with sample inputs and results.
Read the full story here: “Efficient Grid Operation and Maintenance with Simulation Apps”
4. Protect Wind Turbines from Lightning Strikes
Wind turbines are susceptible to lightning strikes, which leads to costly unplanned downtime unless they are properly protected. When designing lightning protection for wind turbine blades, manufacturers know that simple assumptions won’t do. It’s important to be able to predict how much current will flow through the blade and exactly where it will flow, and this requires deep insight into the material behavior and a way of testing the large designs.
At Lightning Technologies, an NTS company in the U.S., designers and engineers use numerical modeling to overcome the challenge of physically testing 70-plus-meter-long blades. For accurate results, they combine simulation with their own material expertise. For instance, the conductivity of carbon (the material wind turbine blades are made of) varies in different directions, and this behavior needs to be accurately represented in the models for the protection to be designed properly. By using experimental values for conductivity, the team at NTS can get realistic visualizations of how the current will flow and then determine how to best protect the carbon blade from burning away due to heating and/or arcing.
Left: Wind turbines struck by lightning. Right: A model of a single wind turbine blade, made of several carbon stacks, showing the current density in the blade.
Read the full story here: “Multiphysics Protects Wind Turbines When Lightning Strikes”
5. Increase the Lifespan of Offshore Wind Farms
Replacing failed transmissions in offshore wind turbines is both difficult and expensive, but such upkeep is required when using mechanical transmissions, where the continual contact leads to wear and tear. The team at Sintex in Denmark has come up with a solution: noncontact magnetic transmissions. When power is transferred via magnetic rather than mechanical forces, the transmission system lasts longer and won’t need to be replaced as often.
To work, the magnetic couplings need to be tailor-made, so as part of the design process, Sintex uses simulation to interchange shapes and materials until the final component meets their customers’ needs. Physical prototyping using magnets is prohibitive from both a financial and time perspective, and because their designs involve multiple physics, COMSOL Multiphysics is the team’s simulation software of choice. To allow colleagues of all development stages as well as sales staff to run their own system tests and design configurations, Sintex also builds custom applications using the built-in Application Builder in COMSOL Multiphysics.
Left: An array of standard magnetic couplings. Right: A 3D model of a magnetic coupling, used for analyzing the temperature distributions of the magnets and magnetic flux densities in the iron.
Read the full story here: “Magnetic Transmissions Increase Lifespan of Offshore Wind Farms”
6. Protect Satellite Systems from Electrical Arcing
Satellite systems fail (partially or completely) if electrical arcing discharge occurs, but it’s hard to predict when this might happen in systems designed for extreme environments, such as space. Preventing destruction from spontaneous electrical arcing requires first finding the critical region where it might occur and then investigating potential triggers. It’s not feasible to reproduce all possible operating conditions in space orbit with experimental studies, but even with simulation there are challenges.
Engineers at the Institute of High Current Electronics (IHCE) in Russia determined that the only way to find self-sustained discharge regions is to numerically simulate the discharge, but that it’s computationally very expensive to do so. In the pursuit of a practical and accurate solution, the team at IHCE used the Application Builder in COMSOL Multiphysics to build a custom simulation application that autonomously partitions and analyzes the device to find the most likely critical regions.
Left: A circuit board that is used in satellite equipment. Right: A critical parameter diagram showing pressure vs. emission for a critical region in the model.
Read the full story here: “Seeking Out Electrical Arcing Regions in Satellite Systems”
7. Develop Custom Capacitors Better and Faster
Capacitor design requirements vary greatly depending on where they will be used, so developers need to consider power specifications, operating temperature ranges, and materials during the design process of custom capacitors. Cornell Dubilier Electronics created high-fidelity multiphysics models to virtually represent the capacitors and then wrapped their models in easy-to-use interfaces (simulation applications). The applications are then used by colleagues in other departments who need to test different design configurations before selecting the best one for the task at hand.
The underlying models developed by the simulation experts are accurate, and the application interface is restricted so the end user only alters a few design parameters that are meaningful to them. This means that colleagues who do not know how to build multiphysics models can still benefit from the results — without sending design specs back and forth to a simulation expert.
Left: Aluminum electrolytic capacitors. Right: One of several simulation applications built and used by Cornell Dubilier Electronics for analyzing capacitors. This particular application is used for studying the effective series inductance of a single-tab film capacitor.
Read the full story here: “Accelerating Custom Capacitor Design with Simulation Apps”
8. Evaluate the Structural Integrity of High-Performance Nuclear Fusion Machines
The MIT Plasma Science and Fusion Center (PSFC) is designing a tokamak to be used as an R&D platform for testing the divertor concepts required for a fusion reactor. The researchers call their proposed tokamak the “Advanced Diverter eXperiment”, or ADX for short, and it would operate at very high magnetic fields, thus increasing the plasma performance to reactor levels. Their process for identifying and understanding the science and technology that will make fusion energy possible entails combining experiment, leading-edge theory, and numerical simulation. One challenge they face is determining if the vacuum vessel can survive worst-case plasma disruption events characterized by high heat fluxes, magnetic fields, and Lorentz forces.
Numerical modeling helped the team find answers to their design questions. They created two models using COMSOL Multiphysics: one for calculating the magnetic fields, eddy currents, and Lorentz forces resulting from a plasma disruption; and another for predicting stress and displacement in the vessel based on the calculations from their first model.
Left: The proposed ADX tokamak design. Right: Simulation results of two tokamak design iterations showing stress and displacement. The top row shows a design with a single fixed boundary and the bottom row shows a design with an additional boundary, where a support block is used as reinforcement.
Read the full story here: “Man-Made Stars: Evaluating Structural Integrity in High-Performance Nuclear Fusion Machines for Power Generation”
9. Develop Custom High-Power Electrical Devices Better and at Lower Cost
Designing custom high-power electrical devices (such as transformers and reactors) often means working with restrictions on size and materials. When customers dictate the specs, each new product request is unique and the design has to undergo rigorous testing before production. One manufacturer of such products, BLOCK Transformatoren-Elektronik in Germany, was looking to cut costs while also improving their services.
Over many years, BLOCK had developed their own formulas for describing magnetic materials and wanted simulation software that would allow them to easily enter these formulas and use them in simulations. They also wanted to utilize high-performance computing (HPC) to get simulation results faster, and ultimately deliver products quicker to the customer. COMSOL Multiphysics was the flexible simulation software they were looking for.
Left: A custom line reactor used for filtering out current spikes and reducing the injection of harmonic currents into the power supply. Right: A 3D simulation of a DC choke showing how it cools using airflow.
Read the full story here: “HPC-Enabled Simulation Aids in the Design of Customized High-Power Electrical Devices”
10. Protect Power Plants from Current Surges and Interruptions
Developing generator circuit breakers for power plants requires coming up with an extremely reliable design (brand new or an upgrade to an existing design) and testing it to make sure it meets standards for commercial use. The earthing switches that ground energized parts of the circuit breaker system also need to be carefully designed and rigorously tested.
For their earthing switches, ABB Group uses a tulip design, and they are always looking to improve their designs. They needed to calculate the total forces acting on the tulip contact, which involves coupled electromechanical behavior. The team found that COMSOL Multiphysics is a very nice tool to combine with empirical testing in their process of analyzing and improving current designs.
Left: The inside of a generator circuit breaker where tulip contacts are used for the earthing switch. Right: A tulip contact model visualizing the current density distribution. The symmetry of the tulip allowed the engineers to reduce the computational cost to 1/8th.
Read the full story here: “Tiptoeing Through the Tulips to Protect Power Plants”
Plus 6 More Power Industry Examples of Using COMSOL Multiphysics®
Read about the ten examples described here and six additional ones in our special edition of COMSOL News for the power industry.
Comments (0)